|
HABITABLE ZONE
|
The Terrestrial planets (Mercury, Venus, Earth, Moon, Mars)
are similar to each other in
mass, diameter, and distance from the Sun.
Because of this, it is expected that
their atmospheres should share many similar qualities.
Despite this, their atmospheres show
significant differences.
Remarks:
- Venus, Earth, and Mars have atmospheres, while there
are only traces of an atmosphere on Mercury and the Moon.
- The Venusian and Martian atmospheres
are predominantly carbon dioxide while the Earth's atmosphere is 78 %
nitrogen and 21 % oxygen
- The Earth is the only planet whose atmosphere contains a
significant amount
of free oxygen; the abundant free oxygen on Earth is a product of
life. There is oxygen in the Venusian and Martian
atmospheres, but it is tied up in carbon dioxide.
- Venus essentially has no water, the Earth has abundant water,
and
Mars shows evidence of water.
- The surface temperatures of the planets
vary wildly from T ~ 900 F for Venus to T ~ 60 F for the Earth.
- The atmospheric masses are in the rough ratio of 100:1:0.01 for
Venus:Earth:Mars (based on their
atmospheric pressures).
There are other differences between the planets, but we
consider the above as the key points for developing an
understanding of the atmospheric evolution of the
Terrrestrial planets.
ORIGIN OF THE ATMOSPHERES
Immediately after formation, Terrestrial planets essentially had no
atmospheres (if they had captured some hydrogen and helium from the
Solar Nebula, it was rapidly lost to space).
Whatever atmosphere
a Terrestrial planet has today was either captured or generated after
the planet formed; the Terrestrial planets have
secondary
atmospheres.
There are two suggestions for the generation of secondary atmospheres:
- Because the planets
formed from the accretion of solid
rock particles, volatile elements were trapped inside of them. Later,
as the interiors of the planetary bodies heated and melted, the volatiles
were released througe volcanic eruptions, outgassing.
- The atmospheres were added to the
planets after they were formed. This could occur either as a slow
capture
from the Solar Nebula directly (not likely), from
material brought in by
the intense Solar Wind from the young Sun, or by comets
(recall the Clementine results for ice on the Moon).
It is not clear which of the above theories are correct.
 |
Outgassing
Current studies of Terrestrial volcanoes show that they do emit large
amounts of volatile materials such as water, carbon dioxide, nitrogen,
and sulfur dioxide (at left is the Santa Maria volcano in Guatemala),
however, it is not clear if enough volatile material can be trapped
during the formation of the planets.
|
For example, consider water. On the Earth, there is enough water to
cover the planet to a depth of around 3.6 kilometers. The oceans thus
contain a mass of water of
Mass ~
1.5x1021 kilograms.
The current rate of outgassing of water from volcanoes is
Outgassing = 1011 kilograms per year
If this rate is typical, it would have taken roughly 15 billion
years
to make the oceans via outgassing. If the rate were only 3 times
higher in the past, then the
oceans could be produced in 4.6 billion years (the age of the
Solar System).
 |
Comets
Comets are roughly half water ice and half rocky material. A 2 km comet
with density 2 grams per cubic centimeter, thus
has mass
M
~ 8x1012 kilograms.
So, roughly 4x108
comets are needed to explain the Earth's oceans.
|
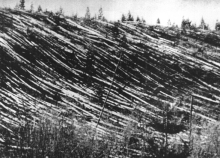 |
There are many hundreds of billions of comets
in the Solar System (in the Oort cloud)
and so, there is an ample supply of comets but, is the
rate of cometary impacts sufficiently large to warrant considering
comets as a viable source for the Earth's oceans?
Based on recent cratering history, the
rate of crater formation by 1 km objects is roughly one every few tens
of thousands of years.
At the current rate, deposition of water
by comets would take tens of
trillions of years. In order for the comet scenario to work,
the cometary rate must have been significantly higher in the
past or there must be a class of small comets (which
are hard to detect), which completely dominates the more typical
observed comets.
ATMOSPHERIC RETENTION
Why does Venus have an atmosphere while Mercury does not?
 |
There are two competing effects which determine whether a planet retains
an atmosphere:
- the strength of the gravitational field at the surface of the
planet (as
measured by the
escape speed of the planet)
- the speed with which the gas particles move around (as determined
by the
temperature and masses of the particles which make up the atmosphere).
|
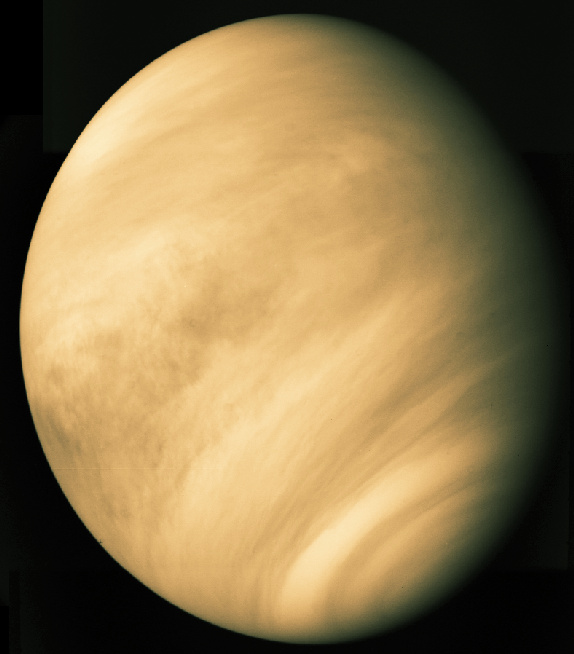 |
There are therefore two important points:
- the mass of the planet is crucial because the escape velocity
depends strongly on
the mass of the planet (via the gravitational force);
the more
massive the planet the higher the escape speed
- the distance of the planet from the Sun because the
temperature of the gas depends
strongly upon how much energy it absorbs from the Sun;
the closer to the Sun the hotter the planet's surface is likely to be,
but there are tweaks to this idea.
EQUILIBRIUM SURFACE TEMPERATURES AND THE GREENHOUSE EFFECT
We now
define the
Equilibrium Temperature.
Assume:
- the planet absorbs heat from the Sun at a certain rate and then
re-radiates this energy at precisely the same rate (hence, the use of
the
word equilibrium).
- the planet radiates like an
idealized creature which is defined to be a perfect emitter and
absorber of
radiation)--a blackbody radiator.
For a planet with an atmosphere, because of the
presence of the atmosphere, not all of the solar radiation
strikes
the planet. Some of it is reflected by the cloud layer and returns to
space.
We measure this effect by defining the Albedo for the planet. The
Albedo, A, is the fraction of the solar radiation which is
reflected to
space. This means that a fraction (1-A) of the radiation reaches the
Earth.
Actual Atmospheic and Equilibrium Temperatures
| Venus | Earth | Mars |
Actual Temp |
>850 F | ~60 F | -60 F --> -70 F |
Eq. Temp |
-20 F | -4 F | -70 F |
The albedo for each planet is 0.65,
0.35, and 0.15 for Venus, Earth, and
Mars, respectively.
For Mars, the equilibrium and actual atmospheic temperatures
are roughly the same while for Venus and Earth,
the temperatures differ significantly. Why?
Because both
Venus and Earth have significant atmospheres and both exhibit the
Greenhouse Effect.
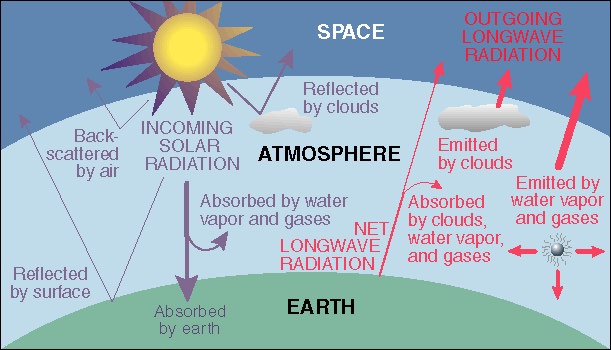 |
|
The Greenhouse Effect
The Greenhouse Effect arises because the
atmosphere of the Earth allow the bulk of the
visible light from the Sun to penetrate
to the Earth's surface. The absorbed sunlight heats the surface of the Earth
causing the Earth to re-radiate the absorbed energy into space. The problem
is that the surface temperature of the Sun is 5,500 Celsius while the Earth
heats to a temperature on the order of -20 Celsius
(with clouds but without an atmosphere), a temperature well below the
freezing point of water! The lower temperature
of the Earth means that less energetic radiation is re-emitted; the re-emitted
radiation falls in the infrared (IR) portion of the spectrum. The problem
is that the atmosphere of the Earth absorbs some of the
re-emitted IR (the Greenhouse gases, for example,
carbon dioxide, water vapor, methane absorb the IR). This traps some of the
outgoing radiation and re-directs it back to the Earth which causes the surface
temperature of the Earth to rise (to ~ 15 Cenlsius).
|
Both Venus and the Earth show Greenhouse Effects; the Earth, however, has a
much milder Greenhouse effect
than found on Venus. The mild
Greenhouse effect is important because it is what makes the Earth
comfortable as we now discuss.
| Faint Young Sun Paradox
The luminosity of the Sun
increases as it has ages; 3.8 billion years ago the Sun
was ~25 % fainter than today.
This is a conundrum because there was liquid water on the
Earth at least 3.7 billion years ago and a
simple argument leads to a prediction
for the Equilibrium Temperature,
Te, which, for the Earth at that time would be below the
freezing point of water, Te = -40 C!. Note that
Te is determined by simply finding the temperature for the Earth
where it radiates exactly the same amount of energy per second as it receives
from the Sun in the absence of clouds and an atmosphere. Further, if we were
to include an atmosphere with the composition of our current atmosphere, the
temperature would rise but would still be less than the freezing point of
water.
|
The answer to the question of
Why do we have liquid oceans? then
requires that our atmosphere in the past must have had a much
different chemical composition than today
so that the Greenhouse Effect could maintain liquid oceans or, perhaps, the Sun
was much brighter in the past than we now believe.
EVOLUTION OF THE ATMOSPHERES OF THE TERRESTRIAL PLANETS
|
|
We consider:
- the current
atmosphere of the Earth; and
- the evolution of the atmosphere of the
Earth.
After this, we consider the atmospheres of Venus and Mars (and
address the question of why the atmospheres of the three planets
are so different).
Atmosphere of the Earth
We first look at the current atmosphere of the Earth. Recall that the
current atmosphere of the Earth has a pressure of 1 bar which is ~ 100 times
larger than Mars and ~ 1 % that of Venus. The
composition of the Earth's
atmosphere is 78 % Nitrogen molecules ad 21 % Oxygen
molecules with
trace amounts of other things, in particular, the greenhouse
gases water, carbon dioxide,
methane, and CFCs.
|
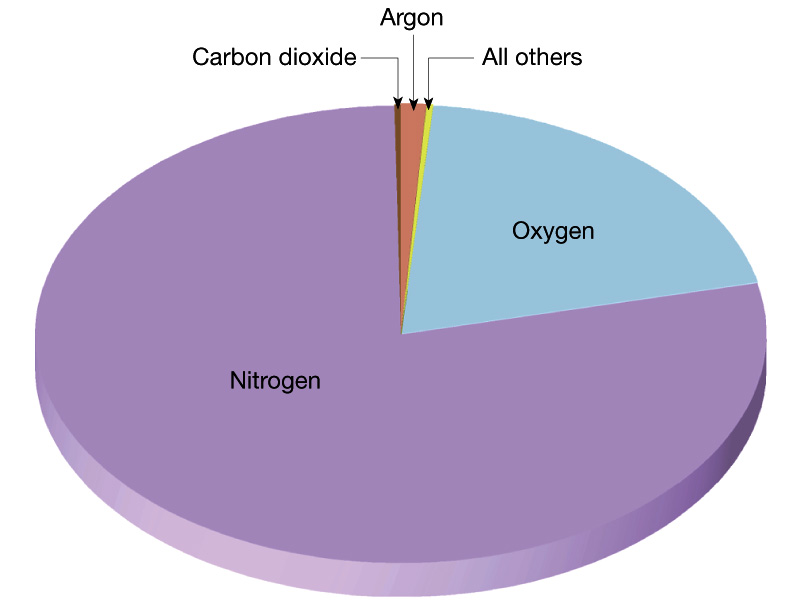 |
The atmosphere is conveniently divided into regions
in terms of how the temperature behaves (whether it is increasing
or decreasing):
|
- Thermosphere:
In the thermosphere, Solar radiation is
able to ionize
(strip electrons off of atoms forming the ionosphere
)
and temperature increases with altitude
(because atoms absorb Solar radiation).
The ionosphere is the layer
which traps radio signals and allows them to be heard around the
world (it is also the layer which gets disturbed and disrupts radio
communication during Solar storms).
- Mesosphere: There are no
strong absorbers of Solar radiation in the mesosphere so
temperature decreases with altitude there.
- Stratosphere:
The next layer of the atmosphere is known as the
Stratosphere and is broken up into layers
composed of different materials (i.e., it is
stratified from which follows its name). The stratosphere is the
layer where Ozone
lives. In the stratosphere, because Ozone
absorbs Solar ultraviolet radiation, temperature increases as
you move upward in altitude through the stratosphere.
- Troposphere:
The lowest layer of the atmosphere, the troposphere is
where atmospheric convection occurs and is the layer which
contains most of the water. The troposphere is the layer
where weather is generated. In the troposphere,
temperature declines with altitude. At the top of the troposphere,
clouds form (because it gets too cold for water to be vapor). This
traps the water in the troposphere, the so-called
Cold Trap. Because the ozone layer lies in the Stratosphere,
the water in the troposphere is shielded from the Solar UV radiation
and is not destroyed by photodissociaion.
|
What Happened to Venus and Mars?
Terrestrial planets (the atmosphere ones) are roughly
the same sizes and same distances from the Sun and yet, they have grossly
different kinds of atmospheres and conditions on their surfaces. Do we have
any ideas as to what leads to the huge differences? Surprisingly, there
may be simple explanations.
Venus, Earth, and Mars
In the beginning, we believe that the material which was outgassed from the
interiors or carried in by comets onto the Terrestrial planets was
similar. That is, the Terrestrial planets started out roughly the same.
Originally, they were dominated by
water, carbon dioxide, sulfur dioxide, carbon monoxide, suflur, cholorine,
nitrogen, molecular hydrogen, sufur, nitrogen, and chlorine, ammonia, and
methane. As the Earth evolved,
oceans formed in the Early Archean
period (the time before 2.5 billion years ago)
as the Earth cooled.
What are the
consequences of the formation of extensive liquid oceans?
On the Earth and Mars:
water vapor likely dominated the early atmosphere, but
because of the temperatures, liquid ocean formed:
|
On Earth, carbon dioxide
dissolved into the oceans, was rained
out of the atmosphere
(and then washed into the oceans), or was directly adsorded into the
rocks and washed into the oceans. Carbon dioxide
deposited into the oceans, settled
and formed sedimentary rocks ===> carbon dioxide was trapped
in the crust! This happened fairly quickly:
|
- On Earth, because of its large mass strongly attracted its atmosphere
gravitationally and because of its magnetic field was able to shield its
atmosphere from the Solar Wind. The combination of these features plus the
strength of the Solar heating allowed the Earth to maintain its
atmosphere. And now, importantly, because of volcanism
(and plate tectonics), a small amount of carbon dioxide and other gases
are returned to the atmosphere (to keep our Greenhouse effect going). We
have been in a fairly stable state for the last 4 billion years or so.
- On Mars a different storyline was followed. The
carbon dioxide was not only deposited into the crust but was also
lost fairly quickly (as was the rest of the atmosphere). Study
of Martian surface features has shown
that the atmosphere of Mars has not changed
greatly for the 3.7-3.8 or so billion years being the inhospitable
world of today. This indicates that
sometime during the first billion or so years of
Mars's evolution, whatever caused Mars to lose the bulk of its
atmosphere, changed Martian climate to what it is today.
There are three primary suggestions:
- gradual erosion by interaction with the Solar Wind
- catastrophic impact of large objects
- evaporation
Recent NASA observations
by Maven (Mars Atmosphere and Volatile Evolution mission)
have shown that the Solar Wind,
the stream of electrically charged
particles continuously blowing outward from the Sun,
was a significant (if not the dominant) contributor to the atmospheric
loss. Unlike Earth, Mars does not have a strong magnetic field to
deflect the incoming Solar Wind particles. Rather, the
charged particles simply crash into the upper atmosphere of Mars
where they collide with and accelerate Martian atmospheric ions. Because
of Mars's small mass, it has a weaker gravitational pull at its
surface, when compared to Earth and Venus, and so has a harder time
holding onto an atmosphere than both Earth and Venus.
MAVEN has observed the Solar Wind's depletion of Mars's atmsophere
bringing scientists closer to solving the mystery of why the
ancient Martian climate is so different from the Martian climate of today.
On Venus:
it is believed that it was too warm for there to be
extensive liquid oceans and the water remained in the atmosphere (Venus is
roughly 30 % closer
to the Sun than is the Earth and receives around twice as much Solar energy).
This meant that all of the carbon dioxide remained in the atmosphere and a
Runaway Greenhouse Effect
ensued. Furthermore, because
water vapor is also a good Greenhouse gas, the early temperature of Venus may
have reached 2,700 F and the surface pressure may have been 300 bars (or
the pressure one would feel living at a
depth of 3 km under the ocean). This is not fun.
What About the Free Oxygen in the
Earth's Atmosphere?
Today, we see that the atmosphere of the Earth contains
~21 % free oxygen. As noted above, at birth there was no
free oxygen. This is good because chemical reactions thought to
produce amino acids are inhibited by oxygen
Where did the oxygen come from?
Oxygen Production:
(i) Photochemical dissociation - breakup of water molecules by ultraviolet
produced free oxygen at ~ 1-2% levels. At these levels, ozone
can form to shield Earth surface from ultraviolet (UV) radiation.
(ii) Photosynthesis - carbon dioxide + water + sunlight ===>
organic compounds + oxygen molecules. Produced by
cyanobacteria (photosynthetic prokareyotes--blue-algae), and
eventually higher plants supplied the rest of oxygen to the atmosphere.
In the Archean period (pre-2.5 billion years ago),
there was little or no free oxygen in the atmosphere
(< 0.001 % of the current level of oxygen,
PAL).
What little oxygen
produced by cyanobacteria
was probably consumed by the weathering process. Only after
rocks at the surface were sufficiently
oxidized could free oxygen remain free in the atmosphere.
During the Proterozoic era (~2.5 to 0.5 billion years ago),
the free oxygen rose to 1 % to 40 % of PAL. Most
of the oxygen was released by cyanobacteria,
which showed a strong
increase in abundance (in the fossil record)
about 2.45 billion years ago. The present level of free oxygen
probably was achieved around ~400 million years ago.
|
|
Evidence from the Rock Record:
(i) iron is extremely reactive with oxygen. If we look at the
oxidation state of Fe in the rock record, we can infer much
about atmospheric evolution.
In the Archean period, we find
minerals in sediments that can only form in non-oxidizing
environments: Pyrite (Fool's gold), and Uraninite.
These minerals are easily dissolved out of rocks under present
atmospheric conditions.
Banded Iron Formation (BIF) - Deep water deposits in which layers of
iron-rich minerals alternate with iron-poor layers, primarily chert.
Iron minerals include iron oxide, iron carbonate, iron silicate, iron
sulfide. BIF's are a major source of iron ore,
because they contain magnetite
which has a higher iron-to-oxygen ratio than hematite. These are
common in rocks 2.0 - 2.8 billion years old, but do not form today.
Red beds (continental siliciclastic deposits) are never found in rocks
older than 2.3 Billion years, but are common during the Phanerozoic time.
Red beds are red because of the highly oxidized mineral hematite
that probably forms by oxidation of other Fe minerals that have
accumulated in the sediment.
Conclusion - amount of free oxygen in the atmosphere has increased with time.
Biological Evidence:
Chemical building blocks of life could not have formed in the presence of
free oxygen. Chemical reactions that yield amino acids
are inhibited by the presence of even very small amounts of oxygen.
Oxygen also prevents the growth of many primitive bacteria such as
photosynthetic bacteria, methane-producing bacteria and bacteria that derive
energy from fermentation.
Conclusion - Today, most primitive life forms are
anaerobic suggesting that the first forms of cellular life
probably also had similar metabolism.
Today, such anaerobic life forms are restricted to anoxic (low oxygen)
habitats such as swamps, ponds, and lagoons.
Atmospheric oxygen built up in the early history of the Earth as the waste
product of photosynthetic organisms and by burial of organic matter away
from surficial decay. This history is documented by the geologic
preservation of oxygen-sensitive minerals,
deposition banded iron formations, and development of continental
"red beds" or BIFs.
Where is Venus's Water?
Because there is no Ozone layer,
the temperature simply decreases as you move up in
altitude around Venus. There is not a water trap and the
water vapor is free to rise up
into the high levels of Venus's
atmosphere where it is broken up by Solar radiation. The hydrogen atoms from
the water then escape to space and the oxygens combine with other atmospheric
gases to form different molecules. Venus thus loses its water. After the
water is lost, the Greenhouse effect eases and the temperature drops to
the mild ~ 800-900 F of today and the pressure drops to 90 bars. |
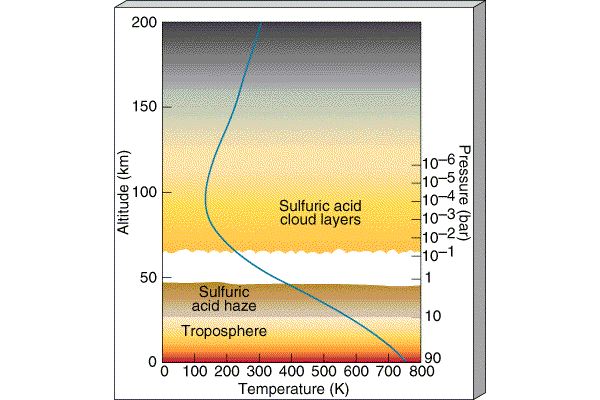 |
THE GAIA HYPOTHESIS
MARS AND WATER
An upshot of the above scenario is that in the past Mars could have had a much
thicker atmosphere and been much more earth-like (there are models which
suggest that the early Mars had an atmospheric pressure of 2 bars!). This
is interesting because, today,
the atmospheric conditions on Mars are such that liquid water cannot
exist on the surface of Mars.
We do see evidence, however, for water on Mars. For example,
there is
in water in the northern residual polar ice cap:
|
The polar caps on Mars have two parts; regions that show
seasonal variations and
residual caps. The seasonal caps are thought to be composed of frozen
carbon dioxide. The residual caps are smaller and brighter than the
seasonal caps and show a very marked north-south asymmetry. The southern
residual cap is frozen carbon dioxide while it is believed that the
northern residual cap is water ice (supported by the observation that water
vapor is observed over the residual cap in the northern summer and the
temperatures of the caps).
|
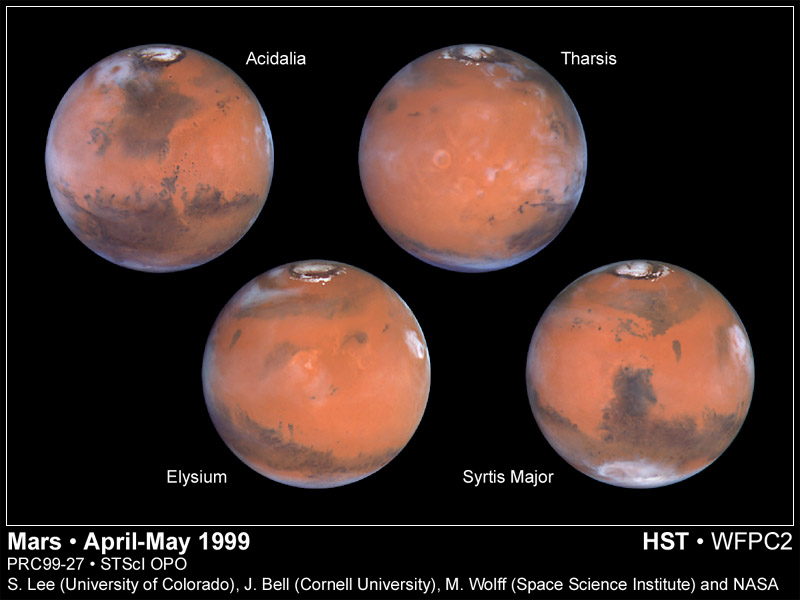
Polar Caps and Clouds |
In addition to the water in the northern residual polar caps, there is
also evidence for water in the low-lying clouds above canyons, and in
large glaciers lying scattered rocky debris:
Clouds Above Canyons
|
Glaciers on Mars
Huge glaciers up to half a mile thick which lie close to the equator of Mars
are thought to be the remnants of an ice age on Mars.
It is thought that the glaciers formed up to 100 million
years ago and represent evidence of climate change on Mars.
Hundreds of glaciers have been identified by researchers using
ground-penetrating radar which allows them to see through the
rocky layers of debris covering the ice. The largest glacier
is 13 miles long and more than 60 miles wide. It could be a
source of water for astronauts on Mars. When the glaciers
formed, Mars' climate was much colder because the angle Mars' spin axis
makes with its orbitl axis was much greater than it is now. This allowed
ice sheets to extend far beyond the polar regions and towards,
possibly even reaching, the Equator.
|
There, presumably, is also a permafrost layer on Mars even today as
implied by
Outflow Channels (large channels which can be up to 100 kilometers and
thousands of kilometers long--likely formed by catastrophic flooding),
"Islands", and
Splosh Craters (oozing mud formed by impacts which melted
the permafrost layer).
The outflow channels and islands were produced by massive floods on
Mars. Presumably what happened was that some event (possibly the impact
of a large object) caused a
rapid, large-scale melting of the permafrost layer which caused floods.
These is ample evidence that water exists on Mars much of it
below the surface which can be
melted and lead to transient flows.
There is also evidence that in the past
water existed in liquid form
on the surface of Mars under quiescent conditions
===> grossly different atmospheric
conditions in the past than presently.
There is thus
evidence that the climate of Mars may have been more Earth-like in the
past than it is today. This leads to the hope that perhaps
life existed on Mars in the past.
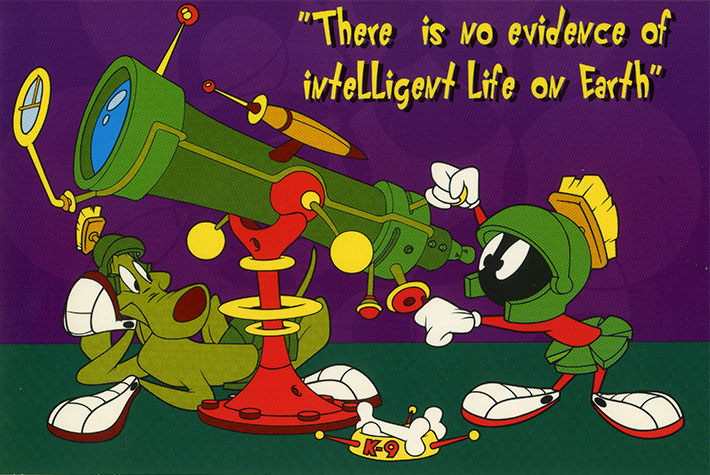
Life on Mars? |
More recent missions to Mars have greatly improved our picture of the Martian
surface and the conditions on Mars today. Curiosity was launched on Nov 26,
2011 reaching Mars on Aug 5, 2012 (landing in
Gale crater).
|
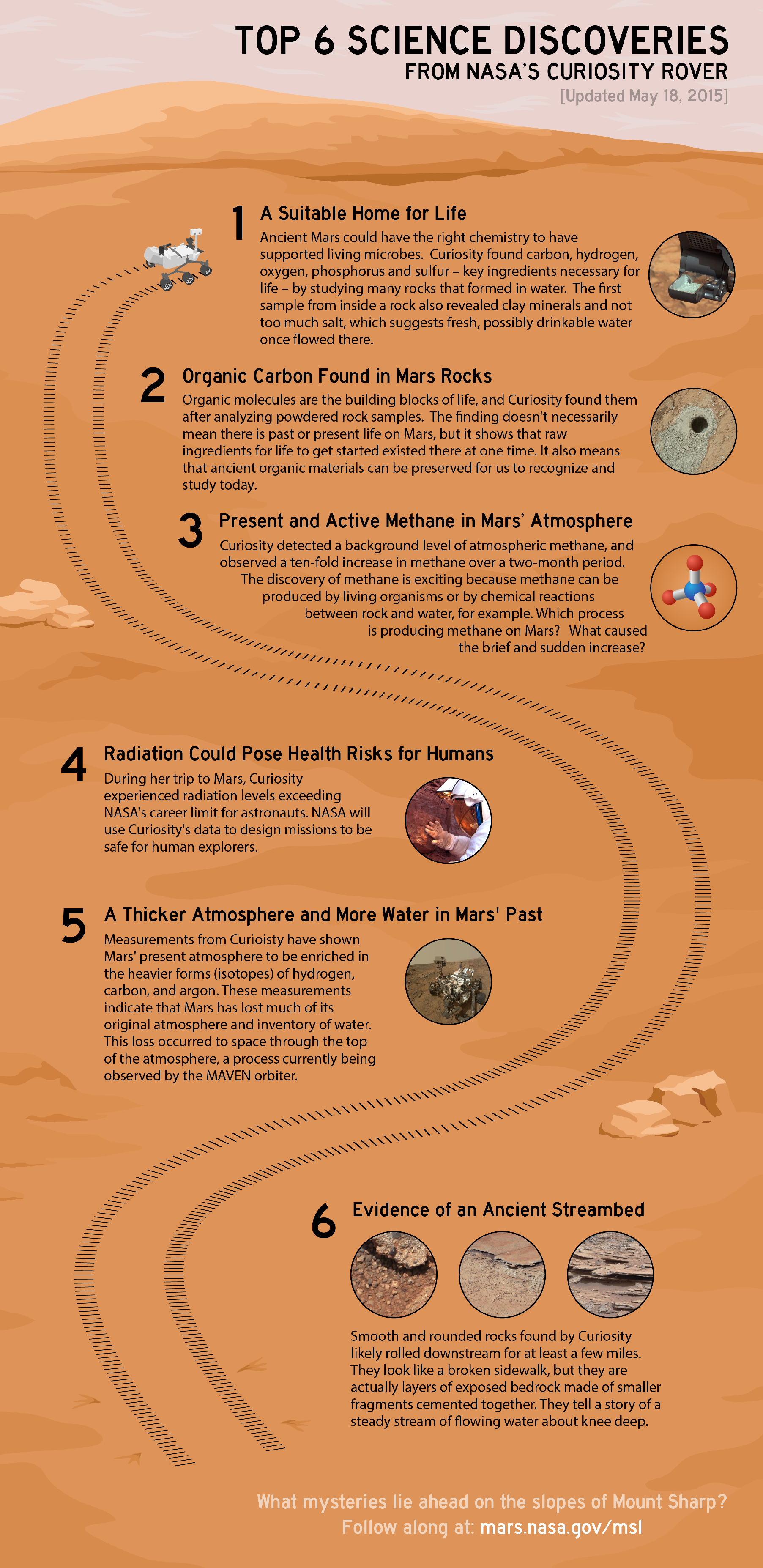 |
Return to Drake Equation Page