|
Atmospheres of the Terrestrial Planets
|
Recall from Topic 5
The Terrestrial planets (Mercury, Venus, Earth, Moon, Mars)
are similar to each other in
mass, diameter, and distance from the Sun.
Despite this, their atmospheres show
significant differences:
- Venus, Earth, and Mars have atmospheres, while there
are only traces of an atmosphere on Mercury and the Moon.
- The Venusian and Martian atmospheres
are predominantly carbon dioxide while the Earth's
atmosphere is 78 %
nitrogen and 21 % oxygen.
The Earth is the only planet whose atmosphere contains a
significant amount
of free oxygen.
There is oxygen in the Venusian and Martian
atmospheres, but it is tied up in carbon dioxide.
- Venus essentially has no water, the Earth has abundant
water,
Mars shows evidence of water.
- The surface temperatures of the planets
vary wildly from T ~ 857 F for Venus to T ~59 F for the Earth
to T ~ -85 F
for Mars.
- The atmospheric masses are in the rough ratio of
100:1:0.01 for
Venus:Earth:Mars.
There are other differences between the planets, but we
consider the above as the key points for developing an
understanding of the atmospheric evolution of the
Terrrestrial planets.
|
In this lecture we consider:
- The origin of the atmospheres
- The retention of an atmosphere
- The Equilibrium Temperature, the
Surface temperature of a planet, and the
Greenhouse Effect
- The Faint Young Sun Paradox
- The Habitable zone concept
I. ORIGIN OF THE ATMOSPHERES
Immediately after formation, Terrestrial planets essentially had no
atmospheres (if they had captured some hydrogen and helium from the
Solar Nebula, it was rapidly lost to space).
The atmosphere
a Terrestrial planet has today was either captured or generated after
the planet formed; Terrestrial planets have
secondary
atmospheres.
There are two suggestions for the secondary atmospheres:
- Outgassing: Because the planets
formed from the accretion of solid rock particles,
volatile materials were trapped inside of them. Later,
when
the interiors of the planets heated and melted, the volatiles
could start to be released through volcanic eruptions.
Current studies of volcanos show that they do release large
amounts of water, carbon dioxide, nitrogen, and sulfur dioxide.
Can volcanism explain our atmospheres?
Consider water. On Earth, we have enough water
to cover the planet to a depth of 3.6 kilometers. This is around
1.5x1021 kg of water.
The current rate of outgassing of water from volcanos is
1011 kg per year.
At this rate, it would take about 15 billion
years to make the oceans via outgassing. If the rate were 3 times
higher in the past, then the
oceans could be produced in 4.5 billion years (the age of the
Earth). Is a tripling of the rate reasonable?
- Capture/Addition: Suppose the atmosphere
was added after formation. This could occur either through comets or
asteroids.
- comets are half rock and half ice and so easily carry enough water.
However, it seems that they cannot not be the sole source for the water
on Earth as the water in comets is different
from the water found on Earth
(there is more heavy water found in the water of
comets than is found in the water of Earth's oceans,
although some comets do contain amounts of heavy water consistent
with that of the Earth's oceans).
Heavy water is a
form of water where the
hydrogen atoms have been replaced by atoms of the isotope of hydrogen known
as deuterium. A hydrogen atom has one proton in its nucleus.
A deuterium atom has one proton
and one neutron in its nucleus.
- Asteroids are roughly 20 % water and so are also potential sources
for our atmosphere and oceans and
they have the right amount of heavy water.
They do, however, have other problems. Asteroids predict that we
would have 10 times more Xenon in our atmosphere than do we.
It is not clear which of the above is correct at this time.
II. ATMOSPHERIC RETENTION
Why does Venus have an atmosphere while Mercury does not?
 |
There are two competing effects which determine whether a planet retains
an atmosphere:
- the strength of the gravitational field at the surface of the
planet as
measured by the
escape speed of the planet.
- the speed with which the gas particles move around is determined by the
temperature and mass of the particles that make up the atmosphere. The
temperature is a measure of the average energy of the particles.
|
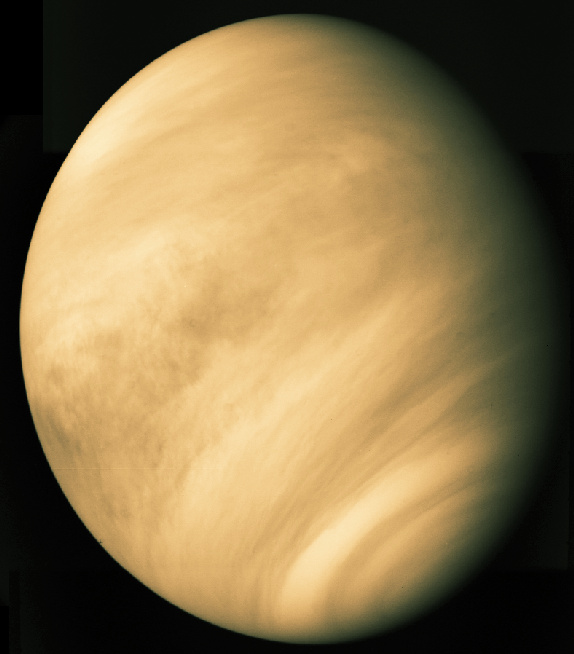 |
There are then two important points:
- the mass of a Terrestrial planet is crucial because the escape
velocity depends strongly on its mass
(via the gravitational force);
the more
massive the Terrestrial planet the higher its escape speed
- the distance of a Terrestrial planet from the Sun because the
temperature of the gas depends
strongly upon how much energy it absorbs from the Sun;
the closer to the Sun the hotter a Terrestrial planet's surface is
likely to be, but there are tweaks to this idea.
Both of the above technically favor Venus, but if you take account of the
atmosphere it gets a little muddied. However, it turns out that even if
you do, it is still true that Venus is able to maintain an atmosphere
while Mercury is not.
III. EQUILIBRIUM SURFACE TEMPERATURES AND THE GREENHOUSE EFFECT
We now
define the
Equilibrium Temperature, Te.
Assume:
- the planet absorbs heat from the Sun at a certain rate and then
re-radiates this energy at precisely the same rate (hence, the use of
the
word equilibrium).
- the planet radiates like an
idealized creature which is defined to be a perfect emitter and
perfect absorber of
radiation--a blackbody radiator.
For a planet with an atmosphere,
not all of the Solar radiation
reaches the surface of the planet. Some of the sunlight
is reflected by the cloud layer back into space.
We measure this effect by defining the Albedo for the planet. The
Albedo, A, is the fraction of the solar radiation which is
reflected back into space.
This means that only a fraction (1-A) of the sunlight reaches
the Earth. The average albedo for each planet is 0.76,
0.35, and 0.15 for Venus, Earth, and
Mars, respectively.
The Te calculation
below takes account of the effects of the clouds (through inclusion of the
Albedo) but it ignores the important effects the atmosphere has on the sunlight
as it travels from the clouds to the surface of the planet.
Actual Atmospheric and Equilibrium Temperatures
| Venus | Earth | Mars |
Actual Temperature |
>850 F | ~60 F | -80 F |
Equilirium Temperature |
-20 F | -4 F | -70 F |
For Mars, the equilibrium temperature and actual atmospheric temperature
are roughly the same while for Venus and Earth,
the temperatures differ significantly in this approximation for
Te. Why?
The atmospheres plays crucial roles. Because both
Venus and Earth have significant atmospheres and both exhibit the
Greenhouse Effect we see the large
differences in the temperatures.
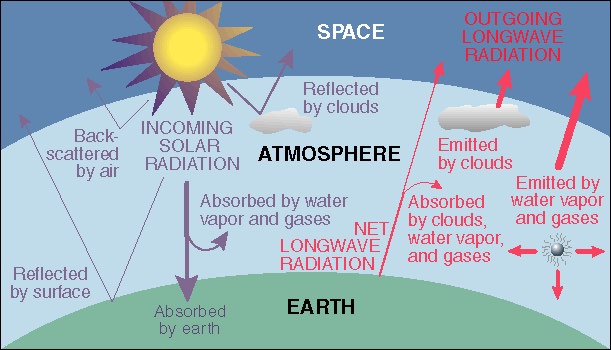 |
|
The Greenhouse Effect
The Greenhouse Effect arises because the
atmosphere of the Earth allows the
visible light from the Sun to penetrate
to the Earth's surface where it is absorbed. The absorbed sunlight heats the
surface of the Earth
and the Earth re-radiates the absorbed energy back to space. The thing
is the surface of the Sun is 10,000 F while the Earth
does not get anywhere near as hot, only heating to around - 4 F initially.
Consequently, the Earth emits
less energetic radiation, infrared radiation, than the visible radiation
the Sun produces. The complication
is that the Earth's atmosphere allows visible radiation to pass freely to
the surface of the Earth but strongly absorbs
infrared radiation not allowing it to escape freely.
The gases that do this are the
Greenhouse gases,
carbon dioxide, water vapor, methane.
This traps some of the
outgoing radiation re-directing it back toward the Earth where it is
again absorbed. This effect raises the
surface temperature of the Earth eventually causing it to rise to ~60 F.
|
Both Venus and the Earth show Greenhouse Effects
. The Earth, however, has a
much milder Greenhouse effect
than found on Venus. The mild
Greenhouse effect is crucial because it is what makes
the Earth habitable.
IV. FAINT YOUNG SUN PARADOX
There were liquid oceans on the Earth as far back as 3.8 billion years ago
based on the ages of sedimentary rocks in Greenland.
Let's see if we can understand why. To do so, we ask the question,
Why were there liquid oceans on the Earth 3.8 billion years ago?
and then answer the question to see if we understand why this is so. Let's go.
|
- First, compare our best model for the temperature of the Earth
to see if it
matches the measured temperature of the Earth (the gray band).
- Calculate the current temperature of the Earth's
atmosphere. Today, our temperature estimate matches the
measured temperature of the Earth.
The heavy black line falls in the gray band.
- Models of the Sun tell us that the
Sun gets brighter
as it ages; 3.8 billion years ago the Sun
was ~25 % fainter than it is today. See red curve.
This is the problem.
- Our model estimate for the temperature of the Earth is now in trouble.
The estimated Te for 3.8 billion years ago is around 0o
F, well below the freezing point of
water. See upper black line.
- In fact, Te only exceeds the
freezing point of water around
1.8 billion years ago.
|
Our conclusion is that the Earth did not have liquid water
3.8 billion years ago, in contradiction of the data. This is the
Faint Young Sun paradox.
To resolve the Faint Young Sun paradox
requires that one (or more) of
our assumptions was wrong.
Which assumption was likely wrong?
- The likely culprit is our implicit assumption that the
composition of the atmosphere does
not change.
- If we relax this assumption, we can resolve the
Faint Young Sun paradox.
- If our atmosphere had more Greenhouse gases
in the past than it does today,
the Greenhouse Effect would be stronger and there could
be liquid oceans.
Models suggest increases in levels
ranging from 10 to 100 times greater than present levels of CO2
work. Are such increases plausible?
V. HABITABLE ZONE
NASA habitable zone
The definition of "habitable zone"
is the distance from a star at which
liquid water could exist on orbiting planets' surface.
Habitable zones
are also known as Goldilocks' zones, where conditions might be just right --
neither too hot nor too cold -- for life. NASA, Apr 2, 2021
Just googled NASA habitable zone
and up popped the above. The definition stated above for
the habitable zone
relies on several assumptions. The first is that
we are going to look for things that are consistent with
Life As We Know It (LAWKI) or
essentially associating life with liquid water. Note that the above
does not take into account all LAWKI, but only
certain types (more on this later).
Also, it is a tad tricky because the defintion assumes that it is only the
distance from the Sun that determines whether a planet has liquid water on
its surface. We know, at a minimum, that we should also include
the effects of clouds and properties of the planet's
atmosphere. Without atmospheres, no Terrestrial planet in our
Solar System would sit in the habitable zone based on the
simplest estimates for their equilibrium
temperatures (no atmospheres).
Also, we know (suspect), that in the first
hundreds of millions of years in the lifetimes of Venus, Earth,
and Mars that they all supported liquid water and so were in the habitable
zone. Today, only Earth has liquid water and so only Earth
sits in the habitable zone.
The habitable zone is an useful concept,
but it should be kept in
mind that it is not an absolute. As stated above, it is more aligned
with the way we used to think. Today, we are more open about where to look
for extra-Terrestrial and extra-Solar life. The
habitable zone is a nice
general way to think about where life is likely to be found in planetary
systems, but it is best to keep an open mind when moving forward
about where extra-Terrestrial life might be found.