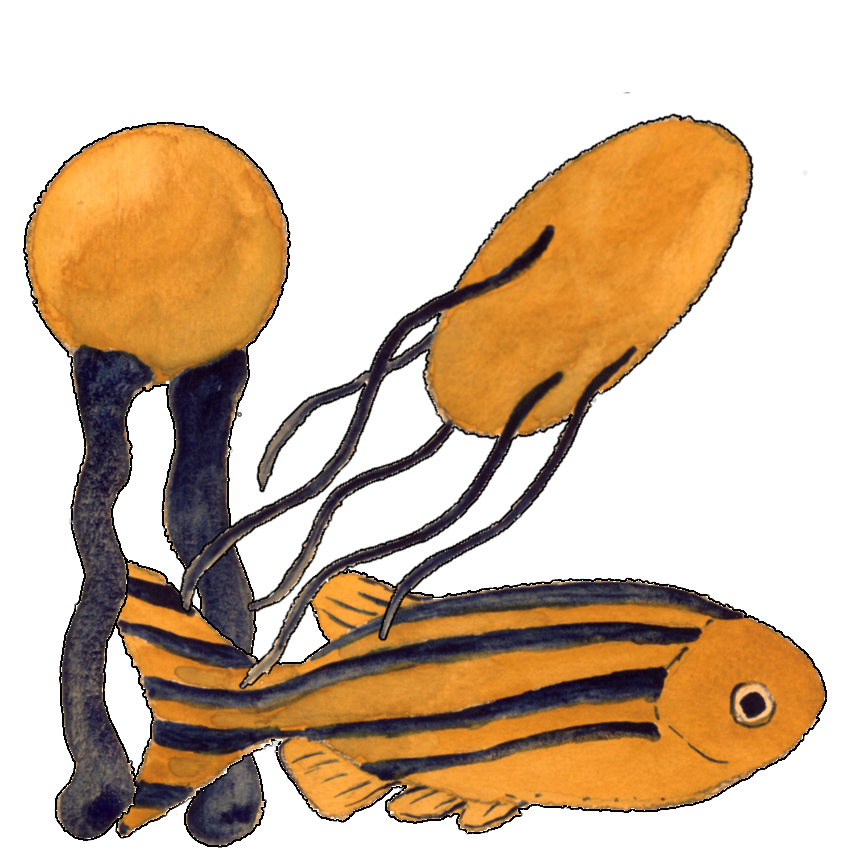
Cellular membranes are remarkable materials: flexible, two-dimensional fluids. Their flexibility is important to the control of shape, as membranes are pinched off into cargo packets, as organelles are formed within cells, and as cells themselves divide. Their fluidity is important to the ability of membrane proteins -- encoded by over a third of the genes in the human genome -- to move and associate with one another as they build structures and perform chemical reactions.
The lipid bilayer forms the underlying architecture of all cellular membranes, and determines the membrane's physical properties. We perform experiments to quantify characteristics such as fluidity and rigidity, often developing new methods involving microscopy, micromanipulation, and particle tracking.Two-dimensional fluidity is one of the most important properties of biological membranes, as it allows lipids and proteins to interact with one another in spatially and temporally dynamic ways. Despite the importance of 2D mobility, its physical underpinnings are poorly understood, and 2D viscosity is not well quantified. We are exploring these topics using microrheological methods -- analyzing the Brownian motion of membrane-anchored particles and lipid domains.
We've developed two new approaches to measuring membrane viscosity. One involves simultaneously examining the rotational and the translational diffusion of membrane-anchored colloidal particles (illustrated below). For a description, see this paper, or this short description. The other involves a method known as "two-point microrheology," in which correlations between the Brownian trajectories of objects in a membrane reveal the viscosity of the embedding membrane. See this paper for more information.
Diffusion of a membrane-anchored tracer pair .
Recently, we've measured how the mechanical tension of a membrane affects membrane fluidity. The three-dimensional analogue of this -- the pressure-viscosity relationship for liquids -- has been studied for over a century. Ours, however, was the first study of this very fundamental relationship for lipid membranes! Surprisingly, we found membrane fluidity to be very insensitive to mechanical tension. See this paper for more information.
The trafficking of cargo in cells involves dramatic transformations of membrane shape and topology by particular trafficking proteins. How do these proteins harness forces, energies, and local material properties to sculpt transport vesicles? How do proteins create curvature? Experiments in our lab seek to illuminate the mysterious mechanics of trafficking with experiments that directly construct, deform, and observe membranes interacting with trafficking proteins.
We have found that Sar1, a protein that regulates vesicle trafficking from the endoplasmic reticulum, lowers the rigidity of the lipid bilayer membrane to which it binds -- by up to as much as 100% as a function of its concentration. This is the first demonstration that a vesicle trafficking protein lowers the rigidity of its target membrane. Our experiments, illustrated below, involve using optically trapped microspheres to create membrane tethers whose properties reveal membrane mechanical properties.
Here's a movie that illustrates a different approach we've taken to studying membrane mechanics (see here for a discussion):
Thermally driven shape fluctuations of a giant lipid vesicle, incubated with the vesicle trafficking protein Sar1. Analysis of the fluctuation spectrum reveals the rigidity of the membrane, which is dramatically lowered by Sar1. From Andrew F. Loftus, Sigrid Noreng, Vivian L. Hsieh, and Raghuveer Parthasarathy, "Robust Measurement of Membrane Bending Moduli Using Light Sheet Fluorescence Imaging of Vesicle Fluctuations," Langmuir 29: 14588--14594 (2013).